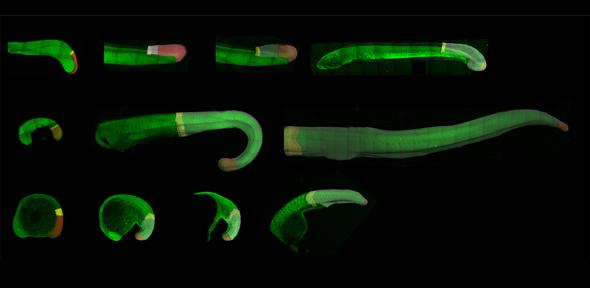
Laboratory of Comparative Developmental Dynamics
Research interests
How is self-renewal and differentiation precisely balanced within stem cell populations to generate well-proportioned tissues during development and growth? To address this fundamental question in biology, I am interested in the development of a bipotent population of stem cells called neuromesodermal progenitors. This essential cell population continues to provide progenitors for both the spinal cord and mesodermal during elongation of the posterior body axis.
Zebrafish have several experimental advantages that allow us to explore the dynamics of neuromesodermal progenitor development at single-cell resolution and in live embryos. We have developed a series of imaging and image analysis techniques:
Firstly, we can quantify gene expression variation between single cells in intact embryos as they exit the tailbud and undergo collective cell fate specification. This makes use of a high signal:noise fluorescent technique termed Hybridisation Chain Reaction.
Secondly, we can follow neuromesodermal derivatives by live confocal imaging and cell tracking to follow their lineage decisions and dynamic cell behaviours in real-time.
Finally, I am collaborating with Scott Fraser’s Translation Imaging Centre at the University of Southern California to develop inducible live reporters of gene expression to follow gene expression dynamics and correlate this with cell fate.
These dynamic and single cell studies in zebrafish will allow for a platform upon which to understand how cell fate specification has been precisely balanced to generate a well proportions body axis.
By extending these studies to amniotes such as mice and chick, we can then begin to understand how a self-renewing stem cell pool has been generated within these organisms to accommodate the increased growth that occurs concomitantly with the generation of the body axis. Unlike the externally developing zebrafish embryo, amniotes such as mice and chickens undergo vast amounts of growth concomitantly with the formation of the embryonic body axis. The posterior body of the mouse embryo increases by approximately 65 times its initial volume during somitogenesis, this is much more that the 4 times increase in volume that occurs in zebrafish. What does this mean for the cell population dynamics between these experimental models? And, given the observed conservation in the requirement for signalling molecules and gene regulatory networks at the population level, how are these mechanisms interpreted differently at the single cell level to accommodate growth?
Finally, a further comparative study across chordates aims to uncover how cellular behaviours have been adapted between different chordate species to generate the diversity in body axis length. In addition to the continued production of progenitor cells from the posterior tip of the elongating body axis (posterior growth), a diverse set of cell behaviours could potentially drive axis elongation. We have shown that in the zebrafish for example, posterior growth is largely absent and that elongation is driven principally by a rearrangement of pre-existing cells. How flexible is the chice of cell behaviours driving axis elongation across chordates? And at what level is this divergence encoded within the system?
For more information see: https://steventonlab.wordpress.com/
Selected publications
- Attardi, A., Fulton, T., Florescu, F., Shah, G., Muresan, L., Lenz, M., Lancaster, C., Huisken, J., van Oudenaarden., A and Steventon, B (2018). Neuromesodermal progenitors are a conserved source of spinal cord with divergent growth dynamics Development: dev.166728 doi: 10.1242/dev.166728
- Steventon BΨ, Duarte F, Lagadec R, Mazan S, Nicolas, JF and Hirsinger H. Species Tailoured Contribution of Volumetric Growth and Tissue Convergence to Posterior Body Elongation in Vertebrates. Resubmitted to Development ΨCorresponding author.
- Steventon B, Mayor R and Streit A. Neural crest and placode interaction during the development of the cranial nervous system. Developmental Biology. 2014. 389 (1): 28-38.
- Theveneau E, Steventon B, Garcia S, Trepat X, Streit A and Mayor R. Chase-and-run between adjacent cell populations promotes directional collective migration. Nature Cell Biology. 2013. 15(7): 763-72.
- Steventon B, Mayor R and Streit A. Mutual repression between Gbx2 and Otx2 in sensory placodes reveals a general mechanism for ectodermal patterning. Developmental Biology. 2012. 367(1): 55-65.
- Steventon B and Mayor R. Early neural crest induction requires an inhibition of Wnt signaling. Developmental Biology. 2012 365 (1): 196-207.
- Steventon B, Araya C, Linker C, Kuriyama S, Mayor R. Differential requirements of BMP and Wnt signalling during gastrulation and neurulation define two steps in neural crest induction. Development. 2009 Mar; 136 (5): 771-9.
- Steventon B, Carmona-Fontaine C, Mayor R. Genetic network during neural crest induction: from cell specification to cell survival. Semin Cell Dev Biol. 2005 Dec; 16 (6):647-54.
Page updated 13 Nov 2018